![]()
Background
Patellar pain is common in both athletic and nonathletic individuals. Among athletes, men tend to present with more patellofemoral injuries, including traumatic dislocations, than women. In the nonathletic population, women present more commonly with patellar disorders.
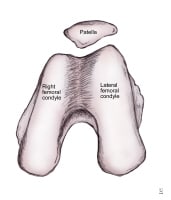
Anatomic morphology of patellar insertion into the intercondylar notch.
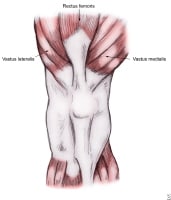
Muscles influencing patellar biomechanics.
Patellofemoral problems are mainly diagnosed by obtaining a thorough history and performing a physical examination. Imaging studies help confirm the diagnosis. Plain radiography is not as sensitive as magnetic resonance imaging (MRI), but it is the least expensive and most readily available modality.
Patellofemoral syndromes are usually the result of biomechanical imbalances of the kinetic chain, with each individual having an optimal joint-loading limit that is dependent on his or her unique skeletal and muscular anatomy, combined with his or her unique neuromuscular patterning. As this limit is surpassed, the patient is at risk for either acute injury, such as patella dislocation, or chronic injury, such as patellofemoral pain syndrome. Therefore, the goal of a rehabilitative treatment program must be to guide the patient toward performing functional activities without surpassing his or her optimal joint-loading limit. Therapy techniques need to be designed around this principle.
In general, surgery is more effective in preventing recurrences of dislocation because skeletal and muscular components of the patellofemoral joint and extensor mechanism are realigned; however, surgery also has risks. In a patient with normal anatomy, surgery should be considered an option after all conservative treatment modalities are unsuccessful. Patients with anatomic abnormalities may benefit from earlier surgical consideration.
Traditionally, several different systems have been used to classify patellofemoral dysfunction. Some were developed from a functional perspective, whereas others were developed from an anatomic viewpoint. This latter perspective was held by Insall and Merchant, who classified patellofemoral dysfunction according to anatomy.
In 1972, Insall proposed a method of classification based on cartilage damage. The 3 categories in his system are normal, damaged, and variably damaged cartilage. In 1986, Fulkerson and Schutzer developed a system based on measuring arthralgias against joint instability to determine the necessity for surgical intervention. In 1988, Merchant created a system of 5 categories for patellofemoral dysfunction, which included acute trauma, dysplasia, idiopathic chondromalacia, osteochondritis dissecans, and synovial plicae.
No standardized and widely accepted method of patellofemoral dysfunction classification applicable for all specialties has been developed. However, for the purposes of rehabilitation medicine, patellofemoral disorders may be loosely divided into 3 categories. These are soft-tissue abnormalities, patellar instability due to subluxation and dislocation, and patellofemoral arthritis.
For excellent patient education resources, visit eMedicineHealth's First Aid and Injuries Center. Also, see eMedicineHealth's patient education articles Knee Pain and Knee Injury.
NextEpidemiologyFrequencyUnited States
Pain of the patellofemoral joint secondary to patellofemoral dysfunction is the most common disorder of the knee. A 5-year study published in 1984 revealed that 25% of all knee issues in a sports injury clinic were of patellofemoral origin. Another study similarly revealed that 1 in 4 runners is afflicted by patellofemoral pain. Whether related to sports or not, 1 of every 4 painful knees has been reported to be the result of patellofemoral dysfunction.
Patellar injury and dislocation are more prevalent in individuals who participate in certain sports and activities. Anterior knee pain is the most common initial manifestation. In order of descending prevalence, soccer players, weight lifters, runners, and shooters regularly report acute knee pain. In addition, studies show soccer players and weight lifters have the most potential for long-term knee pain.
One study reported 52% of 31 soccer players, 31% of 29 weightlifters, 21% of 28 long-distance runners, and 17% of 29 shooters reported knee pain at least once per month.[1] Thijs et al evaluated gait-related intrinsic risk factors for patellofemoral pain in 102 novice recreational runners.[2] The authors findings suggested an increased risk for patellofemoral pain may be due to excessive impact shock during heel strike and at the propulsion phase of running. In addition, Thijs et al believe their results do not support the theory that those at risk for this condition show an altered static foot posture relative to those who are unaffected.[2]
Swimming also places the athlete at risk for knee pain.[3] On the other hand, sports such as tennis are not associated with knee pain. In summary, factors that cause knee pain include the type, amount, and duration of sports activity.
In addition to activity-specific variance, patellofemoral pain displays some variation between the sexes. A study revealed that in the general population, the female-to-male ratio for patellofemoral dysfunction is 2:1. However, in the athletic population, more men than women experience such syndromes. Further, the study revealed acute dislocation occurred more frequently in males and that recurrent dislocation may be more common in individuals whose initial dislocation occurred when they were younger than 15 years.
Patellofemoral disorders are more likely the result of inappropriate activity duration and type as opposed to genetic factors. Aoyagi et al examined the higher prevalence of joint pain of female Japanese individuals living in rural Japan versus female Americans of Japanese descent living in Hawaii.[4] Despite the similar genetic stock, significant differences in prevalence of joint pain were noted. The researchers postulated that environmental factors influencing activity levels and types were responsible.
Similarly, Zhang et al found that Chinese women in Beijing have a higher prevalence of knee osteoarthritis versus American women in Framingham, Massachusetts.[5] Again, this was thought to be the result of the lower activity levels of women living in the United States. In the same study, men from Beijing were found to have a similar incidence of knee osteoarthritis compared with their Framingham counterparts.
International
Nietosvaara et al studied the annual incidence of acute patellar dislocations in Finnish children younger than 16 years.[6] They found an annual incidence of 43 cases per 100,000 children. Over a 2-year period, 72 children revealed patellar dislocations. Of these, 28 (39%) of the knees had associated osteochondral fractures. Of the 28 osteochondral fractures, 15 had capsular avulsions of the medial patellar margin, and another 15 had intra-articular fragments from the patella and/or lateral femoral condyle.[6]
PreviousNextFunctional Anatomy
Soft-tissue elements that affect the patella are the stabilizing capsular and ligamentous structures within which the patella lies. Some ligaments of the knee are continuous with the fibrous capsule surrounding the patella. When injuries occur, all structures are simultaneously affected. These ligaments hold the patella in place during static and dynamic phases.
The synovial capsule, a separate structure, lies deep to the fibrous capsule and may often be damaged.
The regional anatomy of the knee soft tissues is as follows:
Anteriorly: The synovial capsule forms attachments around the peripheral margins of the patella.Laterally: The lateral or fibular collateral ligament is a tough, round cord that attaches proximally at the lateral epicondyle of the femur and distally at the head of the fibula. This ligament transects the tendon of the biceps femoris, and the popliteus tendon runs medial to it. The biceps femoris tendon is very strong, rarely tears, and protects the joint against varus forces. If torn, the biceps femoris tendon usually tears at the distal end, and the peroneal nerve may be injured, resulting in foot drop. In such cases, the head of the fibula is often fractured because the ligament is stronger than the bone. Increased tension from these lateral structures predisposes individuals for lateral patellar tracking and dislocation. Medially The medial or tibial collateral ligament is a flat band extending from the medial epicondyle of the femur to the medial condyle of the tibia. The medial collateral ligament is continuous with the medial meniscus and the capsule of the knee joint. Three medial ligamentous structures provide static restraint to lateral movement of the patella. These were further defined by a cadaveric study conducted by Andrikoula et al.[7] The medial patellofemoral ligament (MPFL) is a band of retinacular tissue that originates at the medial femoral condyle and attaches to the proximal two thirds of the medial border of the patella. This ligament is overlaid by the distal fibers of the vastus medialis obliquus (VMO) muscle, and the authors found that to a variable extent, its fibers merge into the deep aspect of this muscle. The medial patellomeniscal ligament (MPML) attaches the anterior horn of the medial meniscus to the inferior border of the medial patella. The medial patellotibial ligament (MPTL) connects the distal patella to the tibia.The MPFL has been found to be the major medial soft-tissue restraint to patellar lateral displacement. Studies indicate that up to 97% of acute lateral patella dislocations result in disruption of the MPFL. In studies examining in vitro patella subluxation, an isolated release of the MPTL resulted in a 50% increase in lateral displacement. The results of one study on pediatric patients noted that the zone of MPFL injury after primary patellar dislocation was predominantly isolated to the patellar attachment, which is in contrast to previously published literature. MRI findings showed that the anatomic insertion of the MPFL is distal to the physis in 93% of patients and that the MPFL is more likely to be injured at the patellar attachment. These data provide important evidence to assist in surgical reconstruction of the MPFL in pediatric or adolescent patients.[8] Finally, the 2 more distal structures, the MPML and MPTL, provide important secondary restraints.Posteriorly: The oblique popliteal ligament is broad and strengthens the synovial capsule posteriorly. The oblique popliteal ligament originates inferiorly from the medial condyle of the tibia, and it inserts superiorly and laterally to the posterior aspect of the capsule. The posterior capsule is supplemented by the arcuate popliteal ligament, which stretches from the fibular head and splits. Some fibers run medially to insert into the tibial intercondylar area, and other fibers run superiorly and medially to the posterior lateral epicondyle of the femur. Superiorly: The knee joint capsule inserts into the femur proximal to the condylar margins anteriorly and intercondylar line posteriorly. Inferiorly: The knee joint capsule attaches to the articular margin of the tibia and to the fibular head. The capsule opening for the popliteus is located here. The capsule has openings to the bursae and to the popliteus muscle and tendon.
Pain may develop in these periarticular soft-tissue structures as a result of patellofemoral dysfunction, or vice versa. All these structures operate as a functional unit to optimize weight-bearing capacity. These structures decrease joint-reaction forces (JRFs) and form a base of support for the upper body. If one of these structures is altered, a greater risk of patellar injury and dislocation can develop.
The patellofemoral mechanism is very complicated. Patellofemoral malalignment, abnormal patellar configuration, and a previous history of instability increase the risk for anterior knee pain, patellar dislocation, and recurrent dislocations. The risk for symptoms increases when a combination of factors exists.
PreviousNextSport-Specific Biomechanics
Excluding acute patellar trauma, patellar injury and dislocation are the end result of patellofemoral force imbalances. These force imbalances may also result in less dramatic presentations of patellofemoral pain. Deformities of cartilage resulting from arthritis; congenital variants of the patellofemoral joint; imbalances in lower extremity muscular strength and/or firing pattern; skeletal imbalances at the hip, knee, ankle, or foot; and changes of the patellar stabilizing capsular and ligamentous elements may also contribute to the development of patellofemoral pain and/or dislocations.
The patella is the largest sesamoid bone in the body, and it resides within the complex of the quadriceps and patellar tendons, superiorly and inferiorly, respectively. The patella assists in coordinating the forces of these tendons and functions as both a lever and a pulley. As a lever, the patella magnifies the force exerted by the quadriceps during knee extension. As a pulley, the patella redirects the quadriceps force as it undergoes normal lateral tracking during flexion.
The greater the anteroposterior length of the patella, the greater the angle between the quadriceps and patellar tendons, thus decreasing the force generation needed by the quadriceps to support the upper body at any particular angle of knee flexion. One study demonstrated that the patella most significantly increases the moment arm of the quadriceps at 20° of knee flexion. After patellectomy, the moment arm of the quadriceps is obliterated. After patellectomy, one study demonstrated the effectiveness of the quadriceps-patellar moment arm to be reduced by 31% at 0° of flexion, 22% at 30°, 13% at 60°, 12% at 90°, and 10% at 120°.
The quadriceps tendon and the patellar tendon are continuous with each other and work in cooperation. Muscular forces are transmitted in differing proportions to each tendon over the changing angle of the knee as it flexes and extends. At different angles of knee flexion, the quadriceps and the patellar tendons appear to alternate the role of being the primary force transmitter. From 0-20° of knee flexion, the consensus among researchers is that tension in the patellar tendon is greater than in the quadriceps tendon. From 20-50° of flexion, which tendon has more tension is controversial among research findings. From 50° to full flexion, tension in the quadriceps tendon is greater than in the patellar tendon. Theoretically, isolated development of either the quadriceps tendon or the patellar tendon is accomplished by appropriately limiting knee flexion in exercise programs.
The chondral surface of the patella articulates with the trochlear surface of the distal femur, which forms a groove between the medial and lateral femoral condyles anteriorly. The trochlear surface is continuous with the intercondyloid fossa as it extends inferiorly and posteriorly. The lateral aspect of the trochlear surface is more prominent than the medial aspect, and it extends further anteriorly.
The chondral surface of the patella has several divisions. The 3 transverse ridges create 3 roughly equal-sized upper, middle, and lower groups. Two vertical ridges are found on the chondral surface of the patella. The prominent median vertical ridge separates the medial and lateral facets. The facets are at an acute angle to each other, with the prominent ridge acting as their adjoining corner.
These structures form a V-shaped wedge along the transverse plane for the purpose of better insertion into the depression formed by the trochlear groove. The lateral facet is larger in most individuals. The medial facet is further separated into medial and lateral surfaces by a less prominent vertical ridge. The medial surface of the medial facet is sagittally oriented and only makes contact with the femur when the knee is flexed past 90°. Pain at this range of motion (ROM) that is associated with a compressive mechanism, such as increased JRFs, is suggestive of lesions on the chondral surface.
In full extension, the patella does not fit into the trochlear groove but lies over the smooth synovial tissue that overlies the supratrochlear tubercle. The lateral aspect of the tubercle has a smooth, continuous transition with the trochlear groove. The medial aspect of the tubercle is sharply elevated in regard to the trochlear groove. In normal motion, the patella moves superolaterally, riding the lateral aspect of the supratrochlear tubercle so that it makes a smooth translation from the groove to the tubercle.
The cartilage of the patella contacts the trochlear cartilage of the femur to reduce friction during motion of the patellofemoral joint. Gross normal joint motion is along a sagittal plane. This is why examination of the joint at the transverse plane reveals a congruent articulation, whereas the joint along the sagittal plane is incongruent. Good contact at the transverse plane promotes medial/lateral stability, whereas the incongruent sagittal articulation provides more free space for superior/inferior movement.
Compared with the femoral cartilage, the patellar cartilage is thicker, more pliant, and more permeable. In fact, the cartilage of the inner patella at the prominent median vertical ridge is normally the thickest cartilaginous structure in the body, suggesting its role in counteracting tremendous JRFs. These characteristics of the patellar cartilage allow it to sit deeper in the trochlear groove and conform to its shape, allowing for better articulation and distribution of JRFs. However, these actions place a burden on the collagen-proteoglycan matrix of the patellar cartilage and may be the reason for the higher prevalence of patellar cartilaginous lesions compared with femoral trochlear cartilaginous lesions.
JRFs at the patellofemoral joint are directly related to the contraction of the quadriceps.
The stress at the patellofemoral joint can be mathematically defined as the sum JRF divided over the surface area of force distribution. From 0-60°, the surface area of the patella contacting the femur enlarges with increased knee flexion. This provides a larger contact surface area over which to distribute the load as the load is increasing. Beyond 60° of flexion, anatomic studies regarding the contact area have been inconclusive.
The location of contact for the patella and femur vary with different degrees of flexion and joint load. At 0°, no contact occurs; in early flexion, the distal patella contacts the proximal trochlea; at 90° of flexion, the superior aspect of the patella contacts the femur; when flexion is greater than 90°, the contact area returns to the center of the patella; and when the knee is fully flexed, the inner border of the medial femoral condyle is in contact with the small vertical ridge of the medial facet.
Lateral tracking of the patella leads to decreased efficiency of the quadriceps extensor mechanism and increased patellofemoral joint stress. A lateral patellar subluxation of only a few millimeters results in a decreased contact surface area between the patellar and trochlear surfaces as the lateral facet moves closer to the lateral side of the trochlear groove and the distance between the medial facet and the medial side of the trochlear groove increases. The total JRF, initially distributed over both patellar facets, is now completely transmitted to the lateral patellar facet. This increases lateral facet stress and may result in pain, chondromalacia, and the development of arthritic changes.
A summary of forces on the patellofemoral joint follows. They maintain the physiologic positioning of the patella dynamically within the trochlea and extensor mechanism and provide for patella stability and proper tracking.
Static stabilizers: These provide fixed inhibition to lateral translation of the patella and most notably include the MPFL but also include the MPML and MPTL. These 3 structures play a primary role in stabilization during the first 20-30° of knee flexion when the patella has not fully engaged the trochlea. At knee flexion greater than 30°, the geometry of the patella-trochlea interface combined with posteriorly directed force vectors provide most of the stabilization for the joint. Dynamic stabilizers: These are muscular structures and are primarily the quadriceps group. The VMO muscle has been noted to provide a medially directed dynamic stabilizing force on the patella during knee extension. Andrikoula et al's cadaveric study demonstrated that the VMO fibers are at approximately a 40° medially directed angle to the rectus tendon.[7] Weakness of the quadriceps in general, and specifically of the VMO, allows lateral tracking and deviation of the patella. With persistent lateral patellar deviation, lateral structures (eg, distal fibers of the iliotibial band) contract, resulting in further lateral deviation and greater lateral subluxation. Lateral deviation of the patella also results in altered VMO length and/or tension, which may diminish the medially directed force generation of the VMO muscle. The adductor magnus should also be noted with this group because the distal fibers of the VMO often attach to the adductor magnus tendon and strengthening of the adductor group may contribute to the ability of the VMO to provide active, dynamic restraint.
A summary of risk factors for patella subluxation and dislocation is as follows:
Disruption of either of the 2 groups of stabilizers noted abovePatella alta: This is an abnormally high-riding patella and is associated with a long patella tendon. In a healthy knee, the patella is roughly equal in length to the patella tendon. In patella alta, the ratio of the tendon length to the patella body length is increased, placing the patella in an elevated position that delays patella engagement of the trochlea until an increased angle of flexion. This greatly increases the risk for dislocation. Several different methods can be used to measure patellar instability on a true lateral radiograph of the knee. One such method is the Insall-Salvati index. Patella alta is defined as a ratio of patella tendon length divided by the greatest diagonal length of the patella equal to greater than 1.2. Escala et al found 78% sensitivity and 68% specificity for objective patella instability (OPI) for this parameter.[9] Patella tilt: This parameter may be measured on various axial views of the knee. For patella tilt greater than 11°, Escala et al found 93% sensitivity and an odds ratio of 8.7 for OPI.[9] They found this single parameter to be of the highest combined sensitivity and specificity for identifying patients with OPI. Hypoplastic trochlea: This may also be evaluated on a true lateral radiograph. A classification system was designed by Dejour et al and defined 4 grades of dysplasia.[10] Dejour et al also suggested that the so-called crossing sign they introduced was present on 96% of their patients with patella instability. Using a measurement of trochlear groove depth at the Roman arch level, Escala et al found 85% sensitivity and an odds ratio of 7.7 for OPI.[9] Elevated Q-angle: This represents an estimate of potential lateralizing forces on the patella and is affected by several skeletal features. It is the intersection of 2 lines on the anterior aspect of the lower extremity of a standing patient. One line is from the anterior superior iliac spine to the middle of the patella, and the second line is from the tibial tubercle to the middle of the patella; the Q-angle is the angle between these lines. A Q-angle greater than 15° may predispose an individual to lateral patellar tracking and possible dislocations, although some authors report as high as 20° within a patientâs normal range. This topic is explored in more detail in Physical. Genu valgum: This medially directed knee joint may be the result of a valgus femur, valgus tibia, or intra-articular height loss within the lateral compartment of the knee. Genu valgum may be evaluated on an anteroposterior radiograph. Increased valgum increases the tendency for valgus motion of the knee joint with loading and, as such, increases the potential for lateral motion of the patella. Increased femoral anteversion: This increases the internal rotation of the femur and increases the lateral displacement force vector affecting the patella. According to Post et al, this factor is additive when associated with concurrent genu valgum.[11] This factor may be clinically estimated by evaluating relative internal versus external rotation at the hip. However, accurate measurement is best obtained with computed tomography (CT) scanning of the hips. Coxa valga: This also increases the lateral displacement force vector acting on the patella. This skeletal factor increases valgus stress at the knee. Foot pronation: When present, this contributes to the lateral forces on the patella. It can be easily managed with in-shoe orthotics. Lateral tibial tubercle: This moves the pull of the extensor mechanism laterally and thus increases laterally directed forces on the patella. External tibial torsion: This contributes to the lateral placement of the tibial tubercle, thus increasing the effective Q-angle and increasing the lateral displacement vector acting on the patella. Family history of dislocation: This is reported in the literature and may represent a correlation with an underlying biomechanical anomaly preserved among family members. Other: Escala et al identified other radiographic measurements that are indicators of OPI. These include short patella nose ([9] Kinetic chain models
JRFs of the patellofemoral joint are different when studied under closed and open kinetic chain models. When the kinetic chain is closed (eg, leg press, squats), the JRF increases when the knee is flexed 0-90°. To counteract this load, a greater surface area of the patella comes into contact with the femur, effectively dissipating the forces. However, the contact area does not increase as much as the reaction force. Therefore, forces on the contact areas increase during flexion to 90°. Further flexion greater than 90° causes a leveling off or a decrease in the JRFs. After 90° of flexion, the contact of the quadriceps tendon with the trochlear groove further diffuses the load. Irrespective of the cause, JRFs decrease when the knee is flexed 90-120°.
The open-chain model encompasses lower extremity non â weight-bearing exercises such as leg curls and extensions. When the leg is at 0° flexion, the reaction forces of the patellofemoral joint are low because the patella does not contact the femur when the leg is in full extension. Studies have shown widely varying results from 5-25° of flexion. With the knee flexed to 90°, the JRFs increase and the contact area decreases, resulting in very high patellofemoral stress. A study of knee flexion-extension with a 0.9-kg ankle weight showed JRFs are greatest at 36° of flexion. JRFs are lowest at 90° of flexion.
Closed-chain exercises are most protective for the patellofemoral joint when performed at 0-45° of flexion. Open-chain exercises should be performed from 0-5° of flexion and from 90° to full flexion. JRFs should be limited as much as possible during repetitive motion to avoid chondrosis and chondromalacia. In strengthening or rehabilitative exercises for the quadriceps, programs should be designed with open and closed kinetic chain models in mind.
Anatomic variants
When evaluating patients with patellofemoral disorders, the physician needs to consider anatomic variants, which often manifest as bone deformities and would include bipartite patellae. Additionally, the knee joint may be affected by congenital anomalies. Many genetic syndromes involve the knee joint, including congenital patellar aplasia, nail patella syndrome, small patella syndrome, Meir-Gorlin syndrome, RAPADILINO syndrome (RA for radial, PA for absent/hypoplastic patellas and cleft/high-arched palate, DI for diarrhea/dislocated joints, LI for little size/limb malformations, NO for long, slender nose/normal intelligence), and genitopatellar syndrome. A 2005 article by Bongers et al reviews genetic anomalies in greater depth.[12]
PreviousProceed to Clinical Presentation , Patellar Injury and Dislocation